1.IntroductionNoninvasive optical imaging methods such as optical coherence tomography (OCT) need to know the refractive index for high precision measurement. In an OCT measurement, the measured value of microstructure thickness is not its real thickness, but its optical thickness n⋅t, where n and t are the refractive index and thickness of the sample, respectively. Knowledge of the refractive index distribution in tissues is also important for forming a fundamental understanding of the propagation of light in tissue and permits better planning of laser treatment and surgery.1 There are many traditional methods for measuring refractive index such as white-light interferometry, prism dispersion, and planar reflection methods. However, when the object is a turbid medium, such as bio tissue, which has strong scattering, these methods will be invalid. Another reason for precluding these methods is the nonuniformity of thickness and the flexibility of shape of the sample. To solve the problems, new techniques are proposed, which can be classified into two kinds: “focus tracking method” and “optical path shifting method.” 1 2 3 4 5 In some cases, the “full-reflection method” can also be used.6 In this paper, we discuss the principles of the focus tracking method and the optical path shifting method, both based on OCT technique. In the following sections we will show how we modify the methods to be suitable for practice and give some results of our experiment. Finally, the advantages and disadvantages of the two methods are compared. 2.Methods and ResultsOCT is an efficient imaging technique that uses low coherence interferometry to gate out multiply scattered photons.7 The interference signal will be observed only when the optical path length of the sample arm and the reference arm matches to be within the coherence length of the source. When the optical path difference (OPD) is zero, the observed signal reaches maximum. The schematic of an OCT system is shown in Figure 1. Figure 1Schematic of OCT system based on a fiberoptic implementation of a Michaelson interferometer. SLD: superluminescent diode. A/D: analog-to-digital converter. ![]() 2.1.Focus Tracking Method2.1.1.Method DescriptionThis technique for determining the refractive index of bio tissue was originally proposed by Tearney et al.;1 We extend it for multilayer media.2 The procedure can be summarized as follows:
2.1.2.Modifications and Experimental ResultsNoticing that the moving of the sample is equivalent to the reverse moving of the objective, we choose to move the objective instead of the sample. This modification makes it convenient for practical use since it does not need the moving of the sample. Another advantage is that the final expression of refractive index becomes very simple when N.A. is small enough. The reason for this will be explained in the following sections. The modified method can be described as follows:
Figure 2Schematic of light propagation before and after the moving of lens. The dashed line lens represents its position before moving towards the sample. The solid line lens represents its position after moving towards the sample. θ0: the incident angle on the first layer. θm: the refractive angle on top of the layer m of the sample. Δxm: the displacement of the lens; d1, n1: the thickness and the refractive index of the first layer. ![]()
According to the definition of N.A. Since a light “thread” will not change its direction before and after moving the objective, the following equation is obtained From Eqs. (2) and (4) From Eq. (3) Substitute Eq. (6) into Eq. (5) Solving the equation we get With nm known, dm is obtained by Equations (8) and (9) give the refractive index and thickness of layer m of the sample.When θ0=0, i.e., N.A.=0, Eq. (8) can be reduced to and These formulas are very simple and clear. Using this method, in vivo measurement becomes more practicable. At any time, the neglect of N.A. will cause the measured refractive index to be larger than the real value. It can be proven that this deviation increases with the increase of Δym/Δxm. In most cases, the refractive index of bio issue will not exceed 1.5. Therefore, Δym/Δxm or nm 2 will not be greater than 2.25, thus the upper limit of the deviation of neglecting N.A. will be obtained. The smaller the nm 2 is, the smaller the deviation. Figure 3 shows the relationship of nm vs N.A., where Δym/Δxm is set to 2.25. The curve is nearly a straight line when N.A. ranges from 0 to 0.1. In fact, the deviation is less than 0.2 for N.A.=0.1 and less than 0.6 for N.A.=0.2, that is precise enough for the refractive index and thickness measurements of bio tissue. To conclude, if the incident light beams are “thin” enough, so that N.A.<0.2, nm and dm can be calculated by Eqs. (10) and (11). In our experiments, the diameter of the light beam is about 1.5 mm, focal length is about 3.0 cm, N.A. is about 0.01, and so the relationship mentioned above is satisfied.Figure 3Refractive indices calculated by Eq. (8) at different numerical apertures (N.A.), Δym/Δxm=2.25. Note that if we use Eq. (10), the calculated refractive index is 1.5 and will not change at different N.A. ![]() To verify the feasibility of this method, we measured a fused silica with known thickness of 1036.5 μm and the refractive index can be obtained from a handbook.8 The results are shown in Table 1. The measured values are very close to the known value. Table 1
2.2.Optical Length Shifting Method2.2.1.Method and ModificationCompared with the focus tracking method, the optical path shifting method is simpler. This method originated from optical low-coherence reflectometry (OLCR).3 But OLCR is not simple. Moreover, because it uses diverging probe beam to measure transparent media, there is a disadvantage that intense light source should be used for turbid media to achieve distinguishable signals. We modified this method by simplifying its procedure and using focused beam to get a strong signal so that it is suitable for measuring of turbid media such as bio tissue. As mentioned above, only when the optical path length of the sample and reference arm match, will the observed interference signal reach maximum. So it can be used to measure distance precisely. First, a mirror in the sample arm is needed. We place it on the scanning stage. Before the sample is inserted into the optical path, the optical path lengths of reference arm and the sample arm are adjusted to match each other, i.e., the OPD is zero. The position of the mirror in the sample arm is denoted by Z0. Then we place the sample on top of the mirror and move the mirror in the reference arm to new position Z2, as shown in Figure 4, to make the optical path length match again. The OPD is given by where L is the thickness of the sample; L can be achieved by moving the reference mirror from position Z0 to Z1, where another maximum interference signal will be observed. Thus With L and ΔZ, the refractive index is derived byFigure 4Schematic of optical path shifting method. Only parts of the optical path setup are shown in this figure. Z0: the position of the mirror in the sample arm (see left). It also represents the original position of the reference mirror that makes the optical path in the reference arm match the mirror in the sample arm (see right). Z1: the position of the front surface of the sample. It also represents the position of the reference mirror that makes the optical path in the reference arm match the front surface of the sample. Z2: The position of the reference mirror that makes the optical path in the reference arm match the mirror in the sample arm after inserting the sample. ![]() It should be noticed that when using this method, the detecting light beams of both arms must be collimated in order that the measured refractive index is the group index and the measurement precision is high. In the focus tracking method, the difference between the phase index and the group index is neglected.4 Without using the focus lens, the signal-to-noise ratio is low, especially when the sample is a turbid medium such as bio tissue. To solve the problem, we use the lens that has high depth of field. In this case, light in a small region near the focus point can still be treated as collimated. The region has the order of the depth of field. Because the thickness of the sample is thin, usually 1 mm, we can easily get a lens which has the depth of field of above 1 mm. Using this lens, the measured refractive index can be considered approximately as group index and will not lose its precision. To meet this condition, we only need to select a lens which has a proper value of f/d; the depth of field b is determined by the following formula: where f is the focal length, d is the diameter of the light beam. In our experiment, a lens with focal length of 3.1 cm at 1300 nm is used. The diameter of the light beam is 1.5 mm. So the depth of field is 1.4 mm at the wavelength 1300 nm. The sample is cut into slices so that its thickness does not exceed 1 mm, which assures that the measured refractive index is nearly the true group index.2.2.2.Experiment resultsTo verify the feasibility of this method, we measured the same fused silica used in the focus tracking method. The results shown in Table 2 are coincident with the results previously measured by the focus tracking method. Table 2
Then we measured the refractive index of the sarcocarp of a fresh cucumber at 1300 nm. The mean value of four measurements is 1.354±0.002. Changing the source to 850 nm, the mean value becomes 1.361±0.009. These results show that the group index increases when the wavelength decreases. 3.Comparison and DiscussionThe above two methods based on OCT can be used to measure the refractive index and thickness simultaneously. This is very useful when the thickness of the sample is not known or is nonuniform. When using the optical path shifting method, a mirror in the sample arm is necessary for enhancing observed signals. The sample slice must be cut off from its main body to make it suitable to be placed on top of the mirror. So it can only be used in vitro. The focus tracking method does not need a mirror in the sample arm and can be used in vivo. Generally, the reflection on the rough surface of bio tissue is mainly diffuse reflection. For the medium with thickness greater than 1 photon mean free path (MFP), most photons propagate in it and will suffer scattering more than once, which will strongly weaken the observed signals. For a dermis tissue which has absorbing coefficient μa=2.7 cm −1, scattering coefficient μs=187 cm −1 and anisotropy factor g=0.82 at the wavelength of 633 nm,9 if its thickness is about 1 mm, i.e., 300 MFP, the diffuse reflecting light from the back surface will be greatly weakened. This causes the signal from the back surface to be overwhelmed by the noise and it can hardly be detected. It becomes more serious when the intensity of the light source must be limited to some extent. A possible solution to this is to use a high N.A. lens to reduce the size of the focus. Utilizing the confocal condition, a great part of the noise will be gated out. However, its precision will decrease. For a multilayer medium, the problem becomes even severe. Though the focus tracking method can be used to measure the refractive index of a multilayer bio tissue theoretically, no report of successful measurement on this respect has been found. If we have already adjusted the measuring system to a ready state before using the optical path shifting method, the reference mirror only needs to be moved twice to get all the parameters that are necessary to determine the thickness and refractive index of the sample. In one scan, the mirror is moved towards the detector. In another scan, the mirror is moved against the detector. All can be done within several minutes. This also helps to increase the precision of the measurement. If the measuring time is too long, the bio-tissue sample will be dehydrated and denaturalized, which will lead to the change of its physical properties and the measured refractive index will lose its physiological meaning. From the above experiments, it can be seen that the optical path shifting method has relatively smaller error and higher precision. Furthermore, by using the optical path shifting method, we can get the group index of the sample at different wavelengths. In conclusion, the focus tracking method is suitable for refractive index measurement of bio tissue in vivo, but it cannot distinguish group index and phase index. The optical path shifting method can only be used for in vitro refractive index measurement, but its result can be treated approximately as pure group index and is more accurate. The combination of these two methods will greatly enlarge our knowledge scope on the optical properties of bio tissue. AcknowledgmentsThis research was supported by the Basic Research Project of Science and Technology of the Ministry of Education of China under Grant No. 99019. The authors are grateful to Dr. Li Chuanyong for her helpful advice. REFERENCES
G. J. Tearney
,
M. E. Brenzinski
,
J. G. Fujimoto
et al.;,
“Determination of the refractive index of highly scattering human tissue by optical coherence tomography,”
Opt. Lett. , 20
(21), 2258
–2260
(1995). Google Scholar
L. L. Xue
,
C. P. Zhang
, and
X. Y. Wang
et al.;,
“Analysis of the principle for measuring the refractive index of bio-tissue based on OCT technique,”
Acta Scientiarkum Naturalium Universitatis Nankaiensis (in Chinese) , 33
(2), 88
–90
(2000). Google Scholar
W. V. Sorin
and
D. F. Gray
,
“Simultaneous thickness and group index measurement using optical low coherence reflectometery,”
IEEE Photonics Technol. Lett. , 4
(1), 105
–107
(1992). Google Scholar
M. Haruna
,
M. Ohmi
, and
M. Hashimoto
et al.;,
“Simultaneous measurement of the phase and group indices and the thickness of transparent plates by low-coherence interferometry,”
Opt. Lett. , 23
(12), 966
–968
(1998). Google Scholar
G. Song
,
X. Wang
, and
H. Ren
et al.;,
“Simultaneous measurements of the thickness and refractive index of micro-structures in obscure specimen by optical coherence tomography,”
Optik (Stuttgart) , 12 541
–543
(2000). Google Scholar
F. P. Bolin
,
L. E. Preuss
, and
R. C. Taylor
et al.;,
“Refractive index of some mammalian tissues using a fiber optic cladding method,”
Appl. Opt. , 28
(12), 2297
–2303
(1989). Google Scholar
D. Huang
,
E. A. Swanson
, and
C. P. Lin
et al.;,
“Optical coherence tomography,”
Science , 254
(2035), 1178
–1181
(1991). Google Scholar
V. V. Tuchin
,
S. R. Jtz
, and
I. V. Yaroslavsky
,
“Tissue optics, light distribution, and spectroscopy,”
Opt. Eng. , 33
(10), 3178
–3188
(1994). Google Scholar
|
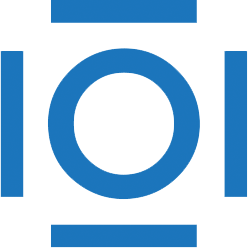
CITATIONS
Cited by 61 scholarly publications.
Refractive index
Mirrors
Tissue optics
Optical coherence tomography
Tissues
Objectives
In vivo imaging